Research
Our Mission
Control of Psychiatric Disease using Genetically Modified Primate Brain
A fundamental goal of human psychiatry is to guide and control emotion and anxiety. Despite its importance, we have not yet elucidated how the brain generates mood and anxiety disorders. Currently, for the treatment of anxiety disorder, obsessive-compulsive disorder and depression, drug medication has been selected, regardless of the severity of the condition. Clinical trials of deep brain stimulation (DBS) acting on the local circuit have started, but the target exists widely in the limbic system, making a systematic understanding remain difficult. It is thus critically important to perform a systematic study to examine the function of the emotional circuit in the non-human primates (NHPs), whose brain structure is homologous to humans.
Many of our decisions involve weighing benefits against costs. The Approach-Avoidance conflict is an essential psychological concept and has been used to characterize anxiety-like behavior and pessimistic decision-making. An optimistic view leads to more approach decisions; a pessimistic view leads to more avoidance decisions. Decisions under approach-avoidance conflict have primarily contributed to characterize the effect of various types of anti-anxiety drugs. Contrary to these advances in neuropharmacology, the underlying neural mechanisms of the approach-avoidance conflict have been less explored. To derive the internal state of optimism and pessimism in the emotional evaluation, we introduced a behavioral paradigm called, “approach-avoidance (Ap-Av) conflict,” and could successfully characterize the value judgment in decision-making. With this task, we could successfully quantify how the subject evaluates the value of reward and punishment. We found that the manipulation of a brain circuit specifically influenced the balance between reward and punishment. We apply computational modeling approach to extract these internal variables, which we cannot directly observe. With this behavioral task, we will newly introduce chemogenetics and optogenetics manipulation to search for the brain region responsible for emotionally abnormal decision-making (Figure 1). We will contribute to the construction of behavioral assays for the ASHBi community, which produces genetically engineered NHPs. For this, we will provide high-throughput assays that can be tractable to anyone by introducing semi-automated in-cage behavioral assays.

Figure 1. Anxiety-related circuitry in the limbic system. Microstimulation of pACC induced pessimistic decision-making under conflict. We will analyze the mechanism of the anxiety-related circuitry by focusing on the cortico-basal ganglia circuitry in the NHPs.
Goals at ASHBi
Research Topic 1: Genetic approach to identify the primate anxiety-related circuits.
We focus on the cortico-basal ganglia circuit to understand how the limbic system induces anxiety and mood disorders. Our previous studies have shown that the downstream region of the limbic cortex in primates contains a striatal striosome compartment, raising the possibility that the anxiety-related circuitry could regulate the nigrostriatal dopamine cells. We will test this hypothesis in primates using genetic techniques to elucidate the mechanism of the generation of anxiety-like states. With the Ap-Av conflict tasks, we will newly introduce chemogenetics manipulation to search for the brain region responsible for emotionally abnormal decision-making (Figure 2).

Figure 2. Chemogenetics manipulation of the dopamine-controlling circuitry
Research Topic 2: Physiological approach to elucidate the interareal communication of the primate limbic systems.
Primates have very large-scale brain network, consisting of massive interareal interactions, which could be acquired through evolution. In particular, the limbic systems of human and macaque monkeys contain specific cellular and network basis such as Von Economo Neuron and neuronal synchronization. We will combine microstimulation and fMRIs to identify the areas with long-distance communication. We will then perform multi-site simultaneous recording to reveal the interareal interaction among the regions in the limbic systems by focusing on their neural oscillations (Figure 3).

Figure 3. Simultaneous recording of spikes and neural oscillations from the large-scale anxiety-related network in the primate limbic systems.
Our Past Research
1. We identified that the pregenual ACC is involved in the generation of an “anxiety-like” state.
We discovered an essential function of the pregenual part of the anterior cingulate cortex (pACC) that projects to the striosome compartment in the striatum. The striosome is an anatomical structure that was histochemically identified by Dr. Graybiel and colleagues in the 1970s. However, very little has been discovered about the function. To understand the role of the striosome-projecting cortex (e.g., pACC), we introduced the Ap-Av conflict task in which the monkey had to decide to accept or reject a combination of good (reward) and bad (airpuff) components (Figure 4). Based on the electrophysiological activity recorded in the pACC, we discovered that the pACC contained neurons that encoded value evaluation (Amemori et al., J Neurosci., 2015). To further substantiate the correlation between these neurons and value evaluation behavior, we directly microstimulated these pACC cells. This microstimulation induced the monkey’s pessimistic decision-making (Figure 4). We further administered an anxiolytic drug while we microstimulated the pACC, and observed recovery from the pessimistic state, supporting the idea that the pACC activation indeed correlated with anxiety (Amemori & Graybiel, Nature Neurosci., 2012).

Figure 4. pACC stimulation induced pessimistic decision. A. Control of decision by pACC microstimulation (blue cross: acceptance of the offer, red square: rejection of the offer, x-axis: offered reward size, y-axis: offered airpuff size). Top: Stim-off block. Middle: Stim-on block. Bottom: The difference between decisions in the two blocks, represented by t-value. The area covered by black contour indicates the offers for which the decision changed. B. For the conflict offer, the pACC stimulation changed their internal value for the punishment. By the stimulation, the monkey overemphasized the punishment. C. The stimulation effect appeared exclusively at the pACC. Sizes and colors of circles indicate percentage of stimulation-induced change in decision (red-orange hues, increased avoidance; blue hues, increased approach) (Amemori & Graybiel, 2012).
Importantly, we recently found that the pACC responses to the Ap-Av conflict were homologous between humans and NHPs, and published it in Biol. Psychiatry (Ironside+, Amemori+, et al. 2019) (Figure 5).

Figure 5. Homologous neuronal mechanism of pACC in humans and NHPs. A. pACC of humans (left) and macaques (right). B. Human fMRI signal responding to aversive offer. C. Distribution of NHP neurons responding to aversive offer, which were dominant in the pACC (*P < 0.05) (Ironside+, Amemori+ et al., 2019).
2. We identified that the cortico-striosome pathway is a causal node of “anxiety” network.
To understand the striosomal function, we used a tracer virus to examine downward projections from the pACC and found that the pACC zone at which the microstimulation induced anxiety-like state indeed preferentially projected to the “striosome” compartment (Amemori, Amemori, et al., 2020) (Figure 6). Further, our group found that the prelimbic (PL) cortex of rats selectively projects to the striosome compartment and that optogenetic stimulation of the PL-striosome pathway specifically affected decision-making under approach-avoidance conflicts. We further showed that stress-induced dysfunction of the striosome circuit caused abnormal choice pattern (Friedman et al. 2015; 2017).
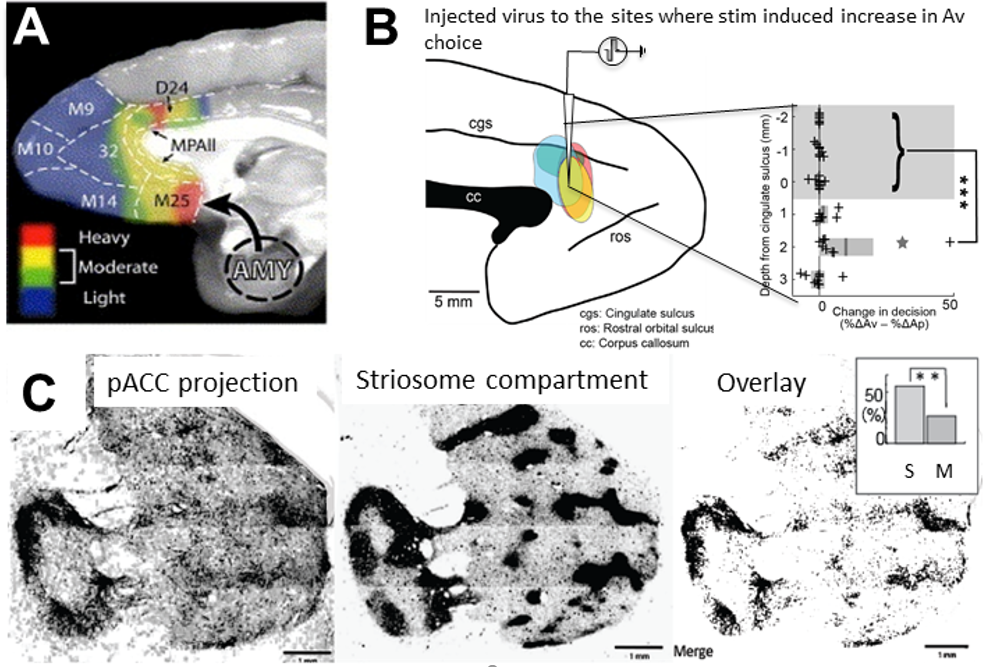
Figure 6. pACC is anxiety-related and projects to “striosome” compartment in the striatum. A. MRI-guided targeting of pACC to stimulate and inject the tracer virus. B. Tracer was injected to the place where the microstimulation induced pessimistic decision. C. Axonal density from the injected sites. D. Striosome compartment in the striatum identified by KChIP1 staining. E. Overlap of C and D. Projection from the pACC local site to the striosome (S projection) was significantly dominant than that to the matrix (Amemori, Amemori et al., 2020).
3. Persistent and repetitive negative thought could be generated by the abnormal striatal activity.
We then sought for the functional differences between the pACC and striatum. Patients with obsessive-compulsive disorders (OCD) often repeat the same decision persistently. We identified that the stimulation of the primate anterior striatum produced an excessive repetition of the negative decision, resembling the symptom of the OCD (Figure 7).

Figure 7. Striatal microstimulation increased repetitive avoidance choices. A. Schematic illustration of the abnormally repetitive thought. B. The sequences of the Ap-Av decisions in the Stim-off (top) and in the Stim-on (middle) blocks. The bottom panel shows the sequence of the reward (red circles) and airpuff (yellow circles) sizes. In the Stim-on block, abnormal repetitive Av choices were observed (Amemori et al., 2018).
4. A computational model of striatal compartments
Why do striosome and matrix exist adjacent to each other in the striatum, although there are almost no direct interactions between them, and even more, the patterns of the input and output regions are different? Why do they form modules and exist in parallel and distributed? What is the role and function of two pathways from striosome to DA cells? From the viewpoint of information processing, the striatum could have many combinations of actors and critics in parallel, and thus can be modeled by modular reinforcement learning. In order to realize modular reinforcement learning in the basal ganglia, how should the striatal compartments divide their roles? To model the dysfunction of striosomes, we compared a modular reinforcement learning system without responsibility signals to the one with responsibility signals (Amemori et al., 2011) (Figure 8A). Then, the modular reinforcement learning system in which the responsibility signal was calculated could quickly switch the strategy in response to a sudden change in the reward position. On the other hand, when the responsibility signal is not calculated, the model could not respond to the change in the environment. Because the model could not switch the learned strategies, it re-learned the strategy from scratch, and it was not possible to change the behavior flexibly (Figure 8B).

Figure 8. Computational modeling of the cortico-basal ganglia circuitry. A. Modular reinforcement learning and striatal modules. B. Computer simulation providing the mechanism of persistence decision-making.
Recent publications (+: equal contribution)
1. Amemori, Graybiel (2012) Nature Neurosci. 15: 776.
2. Amemori, Amemori, Graybiel (2015) J. Neurosci. 35: 1939.
3. Ironside+, Amemori+, et al. (2020) Biol. Psychiatry 87: 399.
4. Amemori, Amemori, et al. (2020) Eur. J. Neurosci. 51: 73.
5. Friedman, Homma, Gibb, Amemori, et al. (2015) Cell 161: 1320.
6. Amemori+,Amemori+, Gibson, Graybiel (2018) Neuron 99: 829.
7. Amemori, Amemori, Gibson, Graybiel (2020) Frontiers Neurosci. 14: 89.
8. Amemori+, Gibb+, Graybiel (2011) Frontiers Hum. Neurosci. 5: 47.
9. Desrochers, Amemori, Graybiel (2015) Neuron 87: 853.
10. Feingold,…, Amemori, Graybiel (2012) J. Neurophysiol. 107: 19